When I wrote the first edition of my high school chemistry textbook (back in 1994), I discussed the impossibility of seeing atoms. Atoms aren’t just small; they are simply too small to be seen, even with the most powerful microscope. That’s because in order for us to see something, light must bounce off it and enter our eyes. Cells on our retina detect that light, send nerve signals to our brain, and our brain interprets the signals to form an image. Since atoms are roughly 1,000 times smaller than the smallest wavelength of visible light, there is no way for light to bounce off a single atom. As a result, we will never be able to see individual atoms, no matter how powerful a microscope we use.
I said that every year to every general chemistry class I taught at the university level, and no one ever questioned my assertion. But homeschoolers think more critically than most students, so the very first year my chemistry course was published, I got a FAX (yes, a FAX) from a student questioning my statement. The student showed me an image like the one below, which was taken with a scanning tunneling electron microscope at IBM. She asked if it wasn’t a picture of individual atoms:
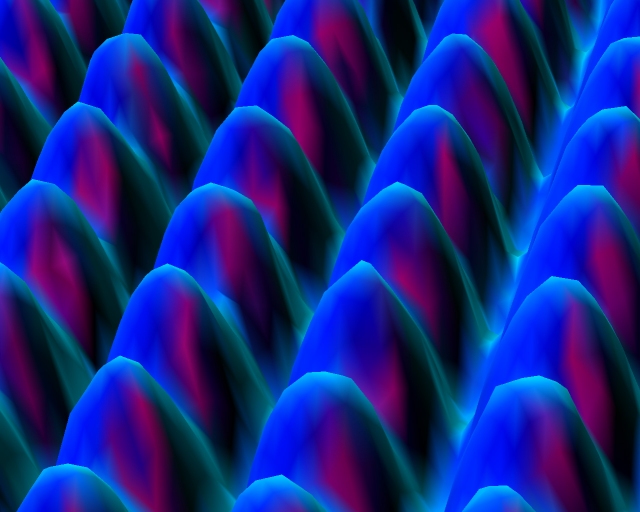
While the image is generally presented as a “picture” of nickel atoms, it is not. It is a graph that represents data that have been processed through a series of equations which govern the behavior of electrons traveling at high speeds. The equations depend on multiple theories, including special relativity and quantum mechanics. I am pretty confident that those theories are mostly true, so I am pretty confident that this is a realistic representation of atoms on the surface of a nickel foil. However, it is not a picture. Light was not used (high-energy electrons were used), and the image is not a direct representation of the nickel surface. It is a direct representation of data that have been processed through mathematical equations that come from several theories.
Recently, I got a question from a teacher who is using my new chemistry course. A student brought him an article that says scientists have been able to take a picture of a single strontium atom. I told the teacher why it is not a picture of a strontium atom, and then over the weekend, I got the same question on my Facebook page. As a result, I decided to write a quick article (well, as quick an article as I can write) about it.
This is definitely a picture, as it is the result of directly detecting light. And, that light is coming from a single atom. That’s the remarkable aspect of the photograph. Because atoms are so small, it is very hard to isolate a single one, but the experimenter who took the picture was able to do just that. However, it is not a picture of the atom. It is a picture of the visible light that the atom is emitting as a result of being excited. When atoms are excited, they must get rid of that excess energy, and one way they can do that is to emit light. The strontium atom is emitting visible light, which is why a picture of it could be taken.
Once again, however, this is not a picture of the atom; it is a picture of visible light being emitted by the atom. After all, the picture shows that the light is purple. If this were a picture of a strontium atom, it would mean that strontium atoms are purple. However, atoms have no color, because they are smaller than the smallest wavelength of visible light.
If you think I am just being pedantic here, consider the picture below:
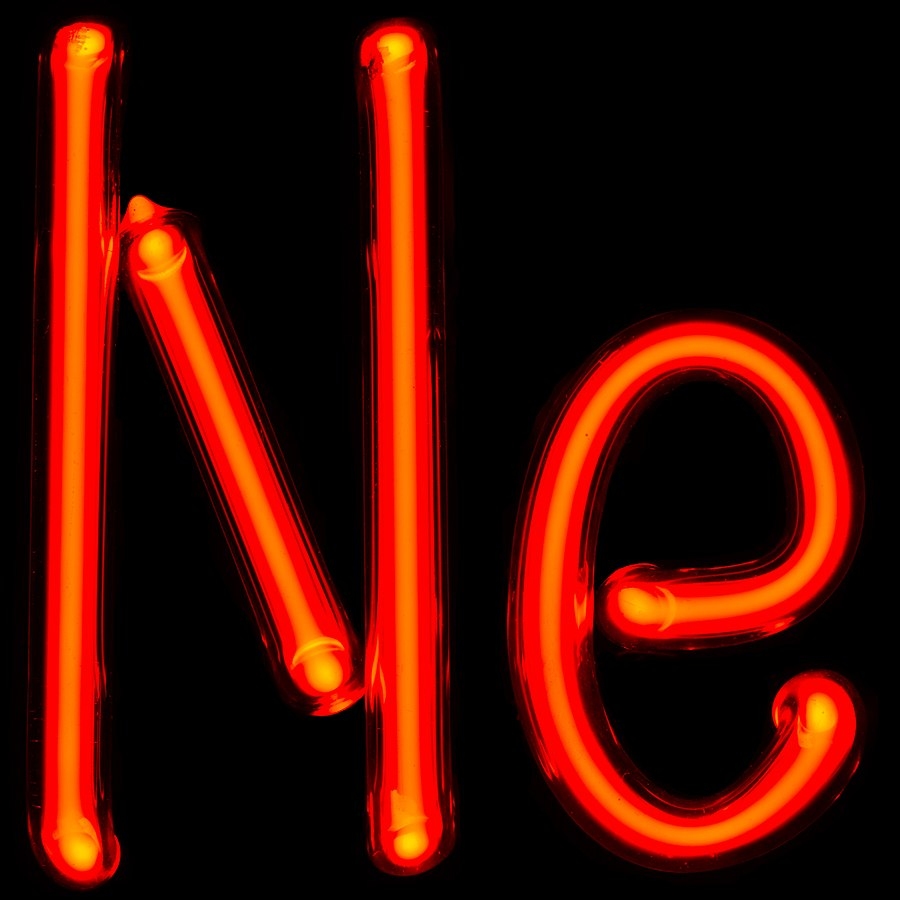
Each tube in the picture contains neon gas, and electricity is being passed through the gas, giving the atoms extra energy. To release that energy, they emit light, and the visible wavelengths that are emitted produce the orange color that you see in the picture. Are you seeing all the individual neon atoms in those tubes? Of course not. You are seeing the light that those excited neon atoms are emitting.
The “picture” of the strontium atom is the same thing, except that there is only one atom emitting light, while there are roughly 100,000,000,000,000,000,000,000 neon atoms emitting light in the picture above.
Thank you Dr. WILE, We trust your science, but we certainly do question things, generally speaking. Our youngest son absolutely loves your science courses, old or new. I have mentioned to our son that he could email you with any questions he may have about the text, or general science questions. It’s great to know how much you care about the education of our kids and are willing to put time into it when needed.
Please keep questioning, Marcy. It is one of the most important things a scientist can do!
Hmm, that’s a strong statement, Jay: “we will never be able to see individual atoms.”
Shouldn’t you say, rather, that “we will never be able to see individual atoms based on the knowledge we have now,” or “It is highly unlikely that we will ever be able to see individual atoms”?
I guess I should say, “I can’t imagine a scenario by which we will ever see individual atoms.” After all, seeing is defined as detecting the light that bounces off something, and since light cannot bounce off individual atoms, I can’t imagine any possible way that we could see them.
Since atoms are roughly 1,000 times smaller than the smallest wavelength of visible light, does that also mean that there are many molecules that can’t be seen, no matter how powerful the microscope?
For instance, a single water molecule is made up of 3 atoms (2 hydrogen and 1 oxygen), that would make it roughly 333 times smaller than the smallest wavelength of visible light, so it would be impossible to see an individual molecule of water no matter how powerful the microscope, right?
It’s a bit more difficult than that, Alaska. In a molecule, the atoms find the lowest-energy point to sit relative to each other. In water, that’s 9.3×10-11 meters apart from one another. There are two bonds like that, arranged at about a 105 degree angle. So roughly speaking, the widest part of a water molecule is about 1.5×10-10 meters. That’s still less than 1,000 times smaller than the smallest wavelength of visible light. So no, we can’t see individual molecules either. However, this does depend a bit on how you define a molecule. Polymers are large molecules that contain repeating subunits. Some of those get large enough to see. They don’t look like a molecule, however, because you don’t see the individual atoms linked together. Vulcanized rubber, for example, can be thought of as just one big molecule.
Hmmm.
While, of course, the various images of lattices of “bumps” aren’t “pictures” of atoms, in that that’s not what they “look like” (since atoms themselves cannot “look like” anything in the sense of human “looking”), I think that the apparatus with the isolated strontium atom is a picture of an atom, in that we can definitely say of the picture, “that’s an atom right there.”
It’s just that, our standard notion of a “picture” assumes the pictured object possesses both spatial features and permanence, neither of which can be represented optically for an atom. Here “seeing” the strontium atom is divorced from seeing ether of those; we cannot use that picture to speak of what a strontium atom “looks like.” But that doesn’t mean we didn’t see it. In particular, when you say in one of the comments above that “seeing is defined as detecting the light that bounces off something,” this is too narrow a definition: We don’t not see stars or glowing iron (or light bulbs!) simply because the light coming from these objects wasn’t scattered from some other source. With some of those the light was scattered within itself internally, but it still comes from the object itself. Iron doesn’t always have to be glowing red, and we still see it, and it’s still iron, whether or not it’s glowing red. Thus I don’t think you can say strontium atoms have to be purple if we claim to have seen the atom itself via the purple light that came from it.
The problem is that “seeing” is philosophically complicated. The strontium atom revealed its location by emitting light; we didn’t see what the strontium atom “looks like,” but did we not see it at all? How much of a difference is there between seeing a thing and seeing where it is? The light came from the strontium atom just like it does from a light bulb, or the neon tubes pictured above. It’s not even removed from direct observation like a particle in a bubble chamber, where we see where it is due to the condensation trail it leaves. A thought experiment that comes to mind: Imagine an extremely light charged lepton with the same charge as the electron—so light that its Bohr radius makes it resolvable by visible light. I’m not sure exactly what we’d see if we had one of these “atoms” made of a proton and one of the light leptons, but if all that’s necessary for “seeing” a thing is that it be as large as the wavelength of visible light, we’d have to say we’d “see” them. So say one of these “atoms” is floating around in a room in its ground state, and we could “see” it. If something then excites it, it may well change color in addition to giving off a photon as it returns to its ground state. Of course, all these things would likely happen too quickly for our eyes to detect, but we’d have this situation: Our “atom” is floating around, visible to us; we see it become excited and de-excite, all the while still visible. Is the light we saw coming from the “atom” due to its transition back into the ground state not “seeing” the “atom”? If it is, we saw the strontium atom, too.
I think this is complicated, but I probably come down on the side that says we saw the strontium atom.
I, too, was always bothered by your textbook’s claims that we can’t “see” atoms; I think I was confused because what you mean when you say we can’t “see” them is that various images of lattices of bumps, which come from data indicating where the atoms are, doesn’t tell us anything about what they look like—there’s no way to “see” an atom by “zooming in” like we do with bacteria and microscopes. I remember thinking from your books that that meant those images of lattices didn’t even tell us where the atoms were, as if it were propaganda to say that science could resolve atoms. Granted, I read your physical science book (I think that’s where I encountered it first) like 16 years ago, so I don’t remember what exactly you said, but I was left with the impression that these “pictures” of atoms were of the same variety of propaganda as the crusade against CFCs—and naturally I was confused when I saw images of quantum corrals, in which atoms were very clearly isolated, if not actually depicted.
I think I’m also okay with saying “that’s an atom” of such images, where it’s understood that what we mean by that is “that’s an atom there.”
Whatever the case, you have certainly succeeded in getting likely hundreds of homeschoolers thinking very intensely about the philosophical nature of scientific images.
It’s an interesting way of looking at the issue (see what I did there?), but I will still have to disagree with you, Jake. Suppose the strontium atom was excited in such a way that it emitted only infrared and longer wavelengths of light. Would we be seeing it then? I could hold up my cheap digital camera, look through the viewfinder, and see a glowing dot where my cheap digital camera detected the strontium atom. I could determine that there is only one, and I could determine its position. However, I am not seeing the atom. I am detecting it. The situation doesn’t change just because the light happens to be visible. Even when the light is visible, we merely detect its position. We don’t see the atom. We see light that the atom has been stimulated to produce.